Methane in Livestock Series
Terragen’s Head of Soil & Plant Health Applications, Dr Paul Scott, has done a deep dive into the world of methane emissions in livestock.
METHANE IN AGRICULTURE
Methane, or CH4, is a problem for agriculture, because it is a greenhouse gas produced in a range of agricultural industries, most notably from ruminant livestock. Agriculture reportedly contributes around 25% towards global greenhouse gas emissions and of all agricultural greenhouse gas emissions, CH4 derived from ruminant animals (including cows and sheep) and their waste constitutes 15-20% of all agricultural emissions.
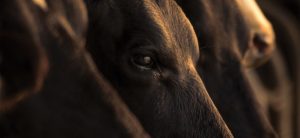
There is some debate regarding the contribution of ruminant-derived CH4 to the total pool of anthropogenic CH4. Some experts argue the CH4 derived from ruminants is part of a biogenic carbon cycle that if animal number remain constant will lead to no net increase in atmospheric CH4. This argument is also supported by CH4 having a relative short half-life of approximately 12 years, compared to the half-life of CO2 of more than 100 years. Of course, this argument does not mean efforts to reduce CH4 emissions from ruminants should be halted.
How is methane generated in the rumen? Bacteria digest animal feed, in the process breaking down the complex carbohydrates, fats and proteins that constitute pastures and grains. The by-products of digestion include volatile fatty acids (VFAs), which are a significant source of energy for the animal. The bacteria, which are collectively called methanogens, use excess hydrogen that is generated during the digestion of feed, to synthesise methane. The bulk of CH4 (around 95%) is expelled from the animal via the mouth through a process known as eructation.
When assessing the methane emissions from ruminant livestock the quantities can be expressed either as absolute or relative methane emissions. Absolute methane yield is the amount of methane emitted per animal without considering diet or animal productivity. Meanwhile, what is more commonly reported across the livestock industry, is relative methane emissions (or methane intensity), which is the amount of methane emitted per kg of dry matter intake or litre of milk produced, or methane emitted per kg of increased carcass weight. Livestock ag tech companies are focused on reducing methane intensity, with published, independent results setting the benchmark for determining the best ways to reduce emissions.
Techniques for measuring methane emissions include:
• Respiration chambers (which measure emissions from individual animals without being affected by external factors; however, are expensive and are difficult to source globally)
• SF6 tablets and canisters (a relative measure of emissions from animals suitable for use on animals in commercial conditions including on pasture)
• GreenFeed system (able to assess individual animals on pasture as they feed; however, animals need to be trained to willingly feed from this apparatus)
• In-direct measurements (e.g. VFAs; are easy to measure in the laboratory; however, are a proxy measure of the likely levels of methane emissions)
• Laser detectors (which are easy to use and able to assess many animals relatively quickly; however, not accepted by some authorities to be as accurate as respiration chambers, GreenFeed system or SF6 canisters)
• Micrometeorological systems (designed to measure “methane clouds” over whole pastures, but this technique is still in early stages of development).
Ag Tech companies aiming to achieve a statistically significant result in reducing methane (p <0.05) can be difficult due to limitations in availability or accessibility to the above equipment and technology. Solutions likely lie in multifactorial approaches including methane mitigators, genetics and diet. No single approach is likely to provide the “silver bullet” to mitigating CH4 emissions from ruminant livestock and their waste, and so integrated approaches using multiple methodologies are most likely the key to future success.
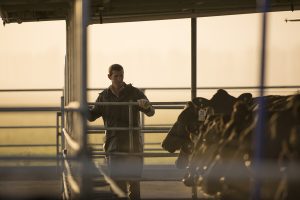
PART TWO – GENETICS
As discussed in Part One of this series, ruminant methane production is the result of interactions between the host animal and the microbiome of the rumen on the ingested feed. Therefore, the genetics of the host as well as the composition of the rumen microbiome will impact the production rate, yield and intensity of the methane produced over the lifetime of the animal.
In the context of mitigating methane emissions from ruminant animals, it is important to note that genetic selection for “elite” animals has been a continuing process for farmers since the origins of livestock domestication. The process of genetic selection for low methane emitting animals has been a relatively recent endeavour as has the recognition that methane emissions from ruminant livestock is a significant contribution to anthropogenic-derived greenhouse gases and the consequential adverse impacts on global climate.
Historically, the process of genetic selection in domestic livestock has been almost exclusively directed towards higher productivity and/or more efficient use of resources (Felius et al. 2014; Weigel et al. 2017). For dairy cows, the traits targeted have included improvements in the quantity and quality of milk production and the efficiency by which animals convert feed into milk. Similarly, feed conversion efficiencies and quantity and quality of carcass have been traits of interest in the beef industry (Arthur et al., 2005; Torres-Vázquez et al., 2018).
In any genetic selection program, the capacity to screen large numbers of animals and have a simple and reliable assay to measure the trait of interest is required. The assay should also not adversely affect the regular animal husbandry practices of a working farm. For productivity traits, genetic selection programs have been relatively straightforward. However, the ability to measure methane emissions using the currently available technologies makes it difficult to screen the large number of animals that are necessary for a valid selection program, based solely on methane measurements.
Many research scientists have looked at the co-inheritance of methane emissions with some of the more easily measurable production traits (Pickering et al., 2015; Smith et al., 2021). While there is not a direct correlation between improved production traits and reduced methane emissions, there is a high degree of co-inheritance for most of the production traits. In other words, the long history of genetic selection programs targeting improvements in production traits (e.g. feed conversion efficiency) has also led to improvements in methane emissions, whether that be methane production (per animal), methane yield (per unit of dry matter intake) or intensity (per litre of milk or kg of body weight).
A more recent innovation with the herd breeding programs of livestock is the incorporation of methane intensity measurements for breeding animals and their progeny. This Sustainability Index is utilised in combination with more traditional trait assessments that rank highly with industry breeding values (e.g., production measures, fertility, resistance to disease) to guide the path of breeding programs. As an industry example of applying this strategy, Dairy Australia – through the initiative DataGene – is providing tools that can help farmers meet the future goals of a more environmentally sustainable industry.
PART THREE – DIET
Awareness around an animal’s gut microbiome leading to its health is at an all-time high. This is as true for humans as much as it is for livestock species. In recent years, a gut’s microbiome population has been linked not only to health and disease of the gastrointestinal tract, but also to the health of organ systems distant from the gastrointestinal tract (Vijay and Valdes, 2022).
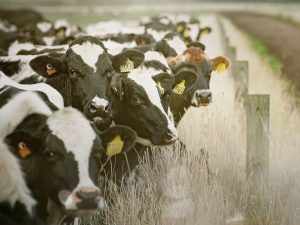
A gut microbiome’s profile is determined by the diet as much as any other factor (e.g. immune status, anatomical features, the microbiome of the mother). Therefore, the constituent microbes (bacteria, fungi and protozoa) will be those that can make best use of the ingested diet as a source of nutrients for their own proliferation. In the context of methane formation in the forestomach of ruminant livestock it is important to note the bacteria responsible for methane biosynthesis (i.e., methanogens) are a taxonomically distinct group from the bacteria that most people would be familiar (e.g., E.coli), instead belonging to the Archaea taxonomic domain. Nonetheless, the methanogenic bacteria are reliant on the digestion of the feed by other resident microbes to produce the precursors necessary for methane formation. Methanogens make use of pools of free hydrogen (H2) formed in the breakdown the animal’s feed in reducing CO2 to form the relatively simple methane molecules, CH4.
The importance of diet to the health and productivity of livestock has long been established. In addition, both the scientific and farming communities have investigated the role diet can play in mitigating methane emissions from ruminants (Beauchemin et al., 2009; Eckard et al., 2010; Martin et al., 2010; van Gastelen et al., 2019), long before the awareness and concern of the general public regarding the contribution of ruminant methane emissions to the overall anthropogenic greenhouse gas emissions.
As mentioned in a previous instalment of this series of posts, the main sources of energy for the ruminant are the volatile fatty acids (VFAs), formed from the feed by the resident bacteria. These VFAs include acetate, butyrate and propionate. Importantly, the relative concentrations of each of these VFAs in the rumen will be determined by both the composition of the diet and the rumen microbiome. Higher concentrations of acetate and butyrate will lead to higher concentrations of residual H2 and as a consequence more CH4. In contrast, if both the diet and microbiome can be re-directed to the formation of relatively higher concentrations of propionate, there will be a smaller pool of residual H2 and so less CH4 formed. This is because in the process of forming propionate much of the residual H2 is consumed.
Some general comments can be made with respect to feed intake and the diet of a ruminant, and how that might impact the levels of CH4 formed in the rumen. Firstly, before considering the composition of the diet, the volume of the rumen and the retention time and rate of passage of the feed through the gastrointestinal tract will determine the amounts of CH4 produced. The larger the volume of the rumen and the slower the passage of feed will allow the methanogenic bacteria to accumulate more CH4. Broadly speaking, forage-based diets lead to higher levels of CH4 produced than grain-based diets. These differences are due to the composition of the respective diets with structural and soluble carbohydrates of forage diets leading to the production of VFAs and H2 more conducive to CH4 production, while the starch, protein and oils found in many grains less conducive to CH4 production. Within these two broad groups of feed, more nuanced differences in CH4 emissions can be seen. For example, the proportion of fibrous carbohydrates as opposed to soluble carbohydrates in forage-based diets will influence the levels of CH4 emissions; the more fibrous carbohydrates the higher the level of emissions.
In assessing which diets lead to the best outcomes with respect to CH4 emissions, it should not be forgotten that the greenhouse gas emissions generated during the production of the forage or grain-based diets need to be included in the overall life cycle analysis of all farm activities.
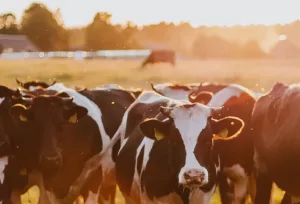
PART FOUR – FEED SUPPLEMENTS
In previous posts, I have described how ruminants generate methane emissions, why these emissions are a significant contribution to increased atmospheric greenhouse gas concentrations, and how some of the historical steps taken to improve the genetics and diets of ruminant livestock have in many instances fortuitously also helped in reducing relative methane emissions from livestock herds.
The final post in this series will address the more recent efforts to discover and exploit feed supplements that specifically target inhibition of methane emissions from the rumen of livestock species (Beauchemin et al., 2022; Hristov et al., 2013; Jeyanathan et al., 2014; Prathap et al., 2021). These feed supplements range from chemicals that inhibit the biochemistry of methane formation by methanogenic bacteria through to direct fed microbials that redirect the substrates for methane formation towards alternative metabolites.
Before listing the different feed types and their means of mitigating methane emissions, there are some important points to note. Firstly, altering the microbiome profile of a ruminant via some of the strategies outlined in this post is a difficult task. The microbiome of a ruminant is established early in the life of the animal. Changing an animal’s gross diet is perhaps the most amenable way to change the microbiome, but simply adding a particular feed supplement is unlikely to make significant changes to the microbiome. Secondly, many of the studies undertaken to determine mitigation potential of feed supplements have been done in vitro under laboratory conditions. In most instances the mitigation potential determined from these in vitro studies are much greater than the mitigation determined in subsequent animal trials. Thirdly, while many of the feed supplements show strong mitigation potential it has also been shown that the mitigation potential is short-lived, perhaps supporting the previous statement that it is difficult to engineer long-term alterations in the established microbiome. Finally, not all the supplements that have been shown to reduce methane emissions also show positive impacts on animal health and productivity. In fact, some feed supplements have been shown to reduce productivity. Further, questions have been raised as to the long-term consequences of feeding some supplements, particularly with respect to effects on animal health and accumulation in the food chain.
The feed supplements that have gained most interest in recent times are 3-NOP and the macroalgae Asparagopsis taxiformis. 3-NOP (3-nitrooxypropanol) is commercially available under the trade name Bovaer® and acts by competitively inhibiting the final enzymatic step in methane biosynthesis. The active ingredient in A. taxiformis is bromoform, and similarly inhibits the biosynthesis of methane. This product is yet to be commercially available as the infrastructure to grow and harvest this seaweed species is not yet established. Two other products that are based on plant extracts as the active ingredient are worth noting because of their recognised credentials by carbon credit certifying organisations. The first, Agolin® Ruminant, is commercially available and contains essential oil extracts from coriander and
clove. It was the first feed supplement to be certified as reducing methane emissions from ruminants, and is recognised by the Carbon Trust UK. The second product, Mootral™, comprises extracts from garlic and bitter orange. The carbon credit status for Mootral™ has been verified and certified by VERRA, a not for profit organisation that manages voluntary carbon market programs.
Direct fed microbials, as feed supplements, present an option that has the potential to be bi-functional, namely that they may be both mitigators of methane emissions and promoters of animal growth and health. The activity of direct-fed microbial products will be determined by their composition, whether comprising a single species or microbial consortia. When considered as a group, the mechanism by which direct-fed microbials mitigate methane emissions might generally be regarded as metabolism that redirects the precursors of methane biosynthesis towards more favourable outcomes. For example, a direct-fed microbial product could contain bacteria that adjust VFA production away from a predominance of acetic and butyric acids to a greater proportion of propionic acid. Direct-fed microbials with other mechanisms of action have been proposed, but at this stage are not proving to be efficacious. For example, the ingestion of bacteria that metabolise methane (i.e., methanotrophs), has been proposed as a strategy to directly eliminate any methane produced in the rumen.
In considering direct-fed microbials and comparing them to chemical-based feed supplements, it is worth noting that if the microbes can establish a sufficient niche within the rumen, then they will have the potential to continue biosynthesis of the active ingredient over an extended period of time, maintaining the effective dose. This contrasts with many chemical feed supplements that have a relatively short half-life in the rumen and will need regular and frequent administration to maintain the effective dose.
At Terragen we have developed MYLO®, a feed supplement based on a consortium of three bacterial species from the lactobacillus genus. Extensive R&D has shown that in dairy cows MYLO® reduces methane emissions and has a positive impact on both animal health and productivity.
REFERENCES
Arthur P. et al. (2005) Reducing the costs of beef production through the genetic improvement of net feed conversion efficiency. Meat and Livestock Australia Project number DAN.075 Final Report ISBN 1 74036 759 6
Beauchemin, K.A., McAllister, T.A. and McGinn, S.M. (2009) Dietary mitigation of enteric methane from cattle. CAB Reviews: Perspectives in Agriculture, Veterinary Science, Nutrition and Natural Resources 4: No. 035. doi: 10.1079/PAVSNNR20094035
Beauchemin, K.A., Ungerfeld, E.M., Abdalla, A.L., Alvarez, C., Arndt, C., Becquet, P., Benchaar, C., Berndt, A., Mauricio, R.M., McAllister, T.A., Oyhantçabal, W., Salami, S.A., Shalloo, L., Sun, Y., Tricarico, J., Uwizeye, A., De Camillis, C., Bernoux, M., Robinson, T., and Kebreab, E. (2022) Invited review: Current enteric methane mitigation options. Journal of Dairy Science 105: 9297-9326 https://doi.org/10.3168/jds.2022-22091
Eckard, R.J., Grainger, C., and de Kelin, C.A.M. (2010) Options for the abatement of methane and nitrous oxide from ruminant production: A review. Livestock Science 130: 47-56. doi:10.1016/j.livsci.2010.02.010
Felius M. et al. (2014) On the history of cattle genetic resources. Diversity 6: 705-750 doi:10.3390/d6040705
Hristov, A.N., Oh, J., Firkins, J.L., Dijkstra, J., Kebread, E., Waghorn, G., Makkar, H.P.S., Adesogan, A.T., Yang, W., Lee, C., Gerber, P.J., Henderson, B., and Tricarico, J.M. (2013) SPECIAL TOPICS – Mitigation of methane and nitrous oxide emissions from animal operations: I. A review of enteric methane mitigation options. Journal of Animal Science 91: 5045-5069 doi:10.2527/jas2013-6583
Jeyanathan, J., Martin, C., and Morgavi, D.P. (2014) The use of direct-fed microbials for mitigation of ruminant methane emssions: a review. Animal 8: 250-261 doi:10.1017/S1751731113002085
Martin, C., Morgavi D.P., and Doreau, M. (2010) Methane mitigation in ruminants: from microbe to the farm scale. Animal 4: 351-365. doi:10.1017/S1751731109990620
Pickering N.K. et al.(2015) Animal board review: genetic possibilities to reduce enteric methane emissions from ruminants. Animal 9: 1431-1440 doi:10.1017/S1751731115000968
Prathap, P., Chauhan, S.S., Leury, B.J., Cottrell, J.J., and Dunshea, F.R. (2021) Towards sustainable livestock production: estimation of methane emissions and dietary interventions for mitigation. Sustainability 13: 6081 https://doi.org/10.3390/su13116081
Smith P.E. et al. (2021) Effect of divergence in residual methane emissions on feed intake and efficiency, growth and carcass performance, and indices of rumen fermentation and methane emissions in finishing beef cattle. J. Anim. Sci. 99: 1-13. https://doi.org/10.1093/jas/skab275
Torres-Vázquez J.A. et al. (2018) Genetic and phenotypic associations of feed efficiency with growth and carcass traits in Australian Angus cattle. J. Anim. Sci. 96: 4521-4531 doi: 10.1093/jas/sky325
van Gastelen, S., Dijkstra, J., and Bannink, A. (2019) Are dietary strategies to mitigate enteric methane emission equally effective across dairy cattle, beef cattle, and sheep. Journal of Dairy Science 102: 6109-6130. https://doi.org/10.3168/jds.2018-15785
Vijay, A., and Valdes, A.M. (2022) Role of the gut microbiome in chronic diseases: a narrative review. European Journal of Clinical Nutrition 76: 489-501. https://doi.org/10.1038/s41430-021-00991-6
Weigel K.A. et al. A 100 year review: Methods and impact of genetic selection in dairy cattle – From daughter-dam comparisons to deep learning algorithms. (2017) J. Dairy Sci. 100: 10234-10250 https://doi.org/10.3168/jds.2017-12954